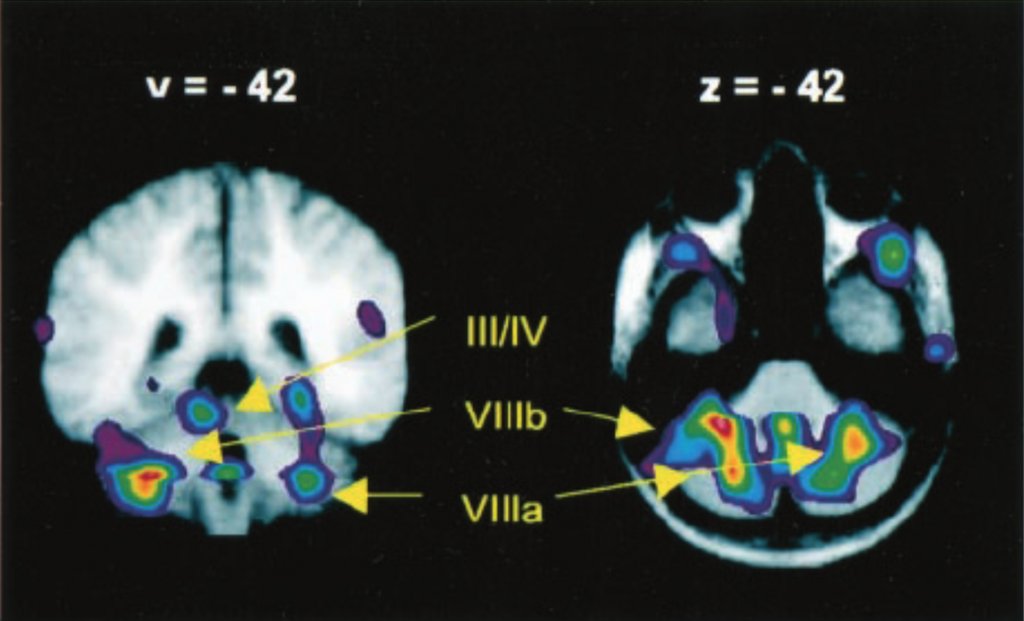
Title of paper under discussion
Dynamic Cortical and Subcortical Networks in Learning and Delayed Recall of Timed Motor Sequences
Authors
Virginia B. Penhune and Julien Doyon
Journal
The Journal of Neuroscience, February 15, 2002, 22(4):1397–1406
Link to paper (free access)
Overview
Using positron emission tomography (PET) researchers from Montreal scanned the brains of volunteers 1) on the first day of learning a tricky finger-tapping rhythm 2) on the fifth day of practising that rhythm and 3) 4 weeks later as they recalled the pattern. The ‘day-one scans’ showed activity (increased blood flow) in the cerebellum, ‘day-five scans’ activity in the basal ganglia, and ‘four-week-later scans’ saw activity in the primary motor, premotor and parietal cortex. These results revealed “a dynamic network of motor structures [in the brain] that are differentially active during different phases of learning and delayed recall”: “early learning” in the cerebellum, “late learning” (or “automatisation”) in the basal ganglia and “delayed recall” distributed between the motor, premotor and parietal cortex.
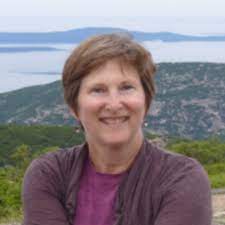
Background
Penhune and Doyon point out that a “large body of literature exists related to motor-skill learning”, studies demonstrating that three brain areas, “motor cortical regions, the cerebellum and the basal ganglia (BG)” are “critically involved in learning skilled movements”. Moreover, these regions are known to be differently active at different stages of learning: cerebellar activity decreases in the course of a motor task being learned, whereas activity in the basal ganglia and motor cortical regions increases. What had not been hitherto studied was “the pattern of activity across these regions for both learning and delayed recall”. In other words, activity in these three brain regions during the first few days of learning a motor task is pretty well understood, but not so the activity in those regions when the task is repeated weeks later.
Therefore the experiment was designed to scan participants’ brains as they first learned a motor task (“early learning”), after 5 days of practice (“late learning”) and then after a 4 week gap with no practice (“delayed recall”).
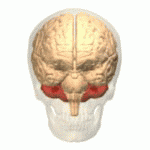
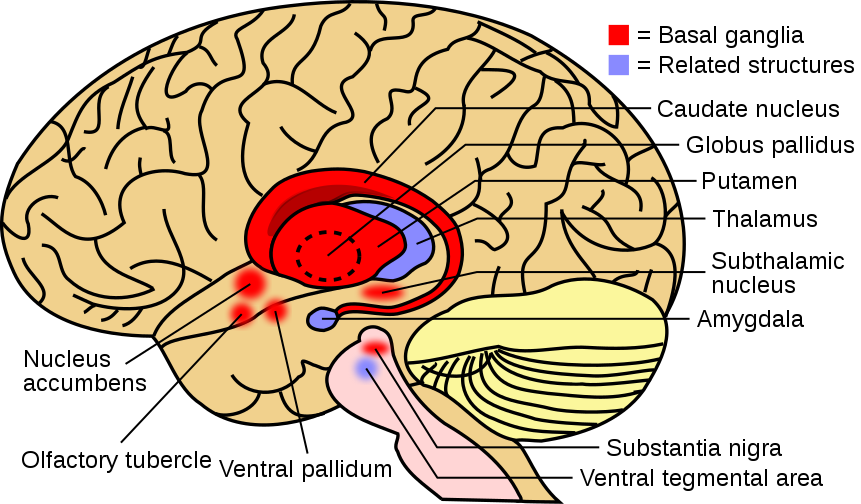
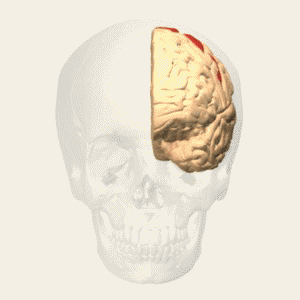
Method
Nine students were paid to take part in the study. Each participant was asked to tap along in time to a “sequence” on a computer screen, the sequence being a square flashing on and off ten times in a long/short note rhythm. Two such “sequences” were presented: 1) a complex rhythm that was relatively tricky to learn (eg ‘short, long, long, short, long, short, short, long, short, long’) and 2) an ‘isochronous’ rhythm that was much easier to learn (eg ‘short, short, short, short, short, short, short, short, short, short’). In the paper Sequence 1 is called LRN, and sequence 2 is called ISO.
On Day One of the training each participant was scanned as they tackled the LRN task and the ISO (control) task. Days Two, Three and Four were further learning days, during which both sequences received regular practice. Then on Day Five each volunteer was scanned again (again tackling both sequences), after which they could go home on the understanding they wouldn’t practise their sequences any more. Then a month later each one returned and carried out the same tasks (LRN and ISO) in the scanner for the last time.
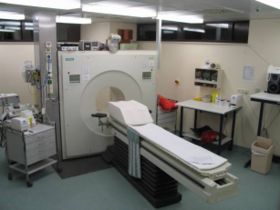
As well as scanning their brains, the researchers were constantly measuring the accuracy of the participants’ sequences, as well as ‘cleanliness’ of the tapping (how consistent was the shortness of the shorts and the longness of the longs, and how well synchronised were the taps with the computer image).
Results
Evidence of improvement
Participants were very quick to learn both tasks, such that the accuracy achieved in both (LRN and ISO) after practising on Day One reached over 90% and remained steady over the next four days and after the four-week gap. However the ‘cleanliness’ measurements revealed continuous learning. Synchronisation accuracy, for both tasks, improved steadily over the 5 days, and remained steady over the 4-week gap. Consistency of tap length was already excellent on Day One for the ISO task, and didn’t go on to improve significantly, whereas for the LRN task it started relatively low on Day One and went on to improve steadily over the 5 days.
Brain Activity
On Day One PET scans taken during the tricky-to-learn LRN sequence compared with during the easier-to-learn ISO sequence revealed more activity in the cerebellum (as well as hippocampal region and extrastriate visual cortex):
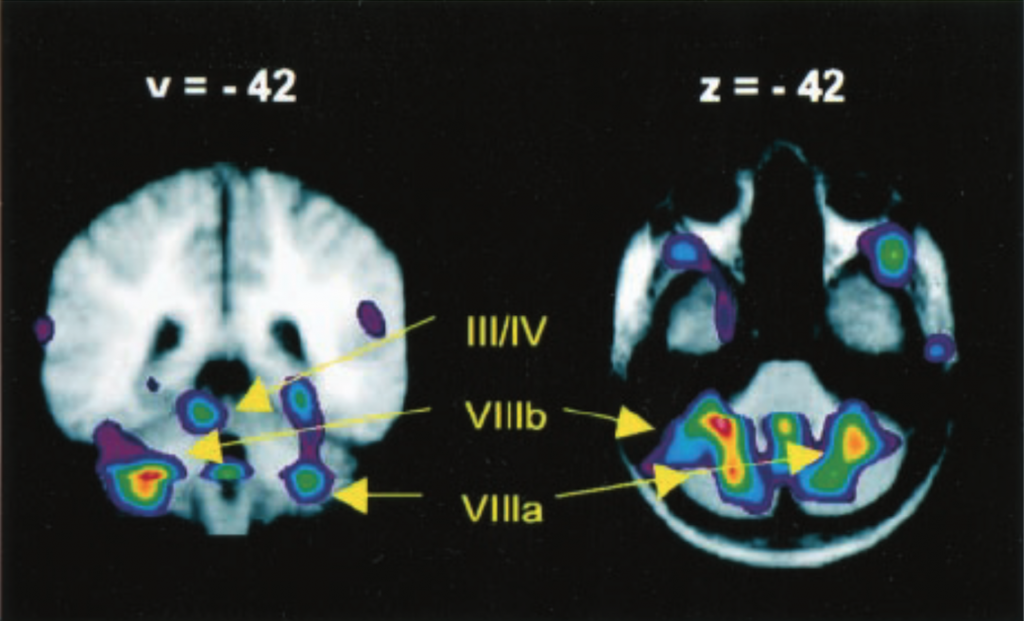
Scans taken during the LRN sequence on Day Five, when compared with those taken during the LRN sequence on Day One, revealed greater activity in the right basal ganglia (as well as medial and orbital frontal cortex):
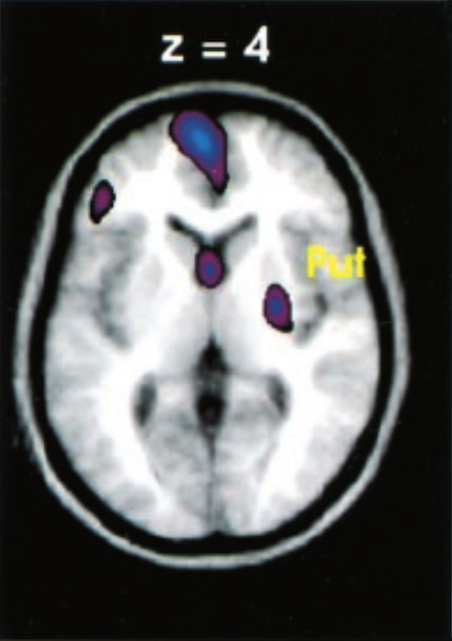
And scans taken during the “delayed recall” of the LRN sequence after 4 weeks, when compared with the those taken during the same sequence on Day Five, revealed greater activity in the left primary motor cortex, the premotor cortex and the inferior parietal cortex:
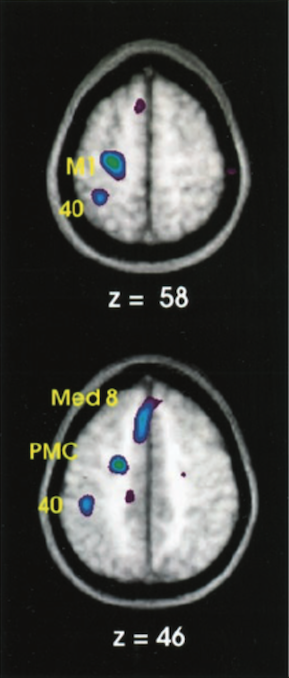
Further statistical analysis confirmed these broad findings. On any day the cerebellum was generally more active during LRN sequences compared with during ISO sequences, but with a significant decrease in activity during those LRN sequences as the practice days went by. The basal ganglia were more active during the LRN sequence on Day Five compared with the same sequence on Day One, but there was no such gradient regarding the ISO sequences. And the primary motor cortex, premotor cortex and parietal lobe areas showed greater activity during the LRN sequence 4 weeks on than during the same sequence on Day Five.
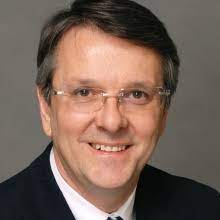
Discussion
Pentane and Doyon conclude “These results demonstrate a network of cortical [referring to the primary motor cortex, premotor cortex and parietal lobe] and subcortical [referring to the basal ganglia and the cerebellum] structures that contribute differentially to the early and late phases of motor learning and to delayed recall. Early learning showed extensive activation of the cerebellar cortex [part of the cerebellum]. After 5 days of practice, cerebellar activity decreased and greater activity was observed in the BG [basal ganglia] and frontal lobe. At delayed recall, significantly greater activation was seen in M1 [primary motor cortex], PMC [premotor cortex], and the parietal lobe, with no significant activity in the cerebellum or BG.”
Drilling further into the data, they reveal that over the course of Day One the participants showed a marked improvement in ‘cleanliness’ of tapping during the LRN sequence; this concurs with the finding of heightened activity in the cerebellum, a brain area known to mediate the creation of movement ‘synergies’, timing, sensorimotor integration and error correction.
The Day Five data showing increased basal ganglia activity is consistent with that area’s known role in the “performance of well learned sequences”, in the “reproduction of simple timed sequences”, and in motivation, assuming that the newly expert performance on Day Five was perhaps “rewarding” for the participants.
The data from ”delayed recall” after 4 weeks, showing increased activity in primary motor cortex, premotor cortex and parietal cortex, is consistent with activity seen the same areas during “overlearned” motor skills such as typing and writing.
In short, conclude the authors, this experiment “reveals a dynamic network of cortical and subcortical structures active during early and late motor learning and at delayed recall.”
Coda
Columbia Symphony Orchestra, conducted by the composer